Where Do Galaxies Come From?
Cosmic harassment, stripping, strangulation, and cannibalism.

Courtesy of NASA/ESA/K. Kuntz/JHU/F.
Bresolin/University of Hawaii/J. Trauger/Jet Propulsion Lab/J.
Mould/NOAO/Y.H. Chu/University of Illinois/Urbana/STScI
We live in a galaxy, the Milky Way,
and when we peer out with our augmented telescopic eyes, we see galaxies
everywhere we look. We see big ones, small ones, richly detailed
spirals, and bulbous ellipticals. We see loner galaxies off on their
own, galaxies that have captured smaller ones that wandered too close,
and massive clusters with hundreds to thousands of galaxies swarming
around one another. We are surrounded by galaxies in all directions,
billions of them. So you would think we’d have a pretty good idea of how
they got there. You’d be wrong. For as much as we’ve deduced about how
the universe was born and evolved, we know surprisingly little about
these ubiquitous structures.
Let’s start with what we do know. Galaxies started forming in the
very early universe, just a few hundred million years after the Big
Bang. The first ones were born out of halos of dark matter—a substance
that astronomers still don’t understand but that interacts with visible
matter (atoms like those in you and me) solely through gravity. These
were not halos in the angelic sense, but rather quasi-spherical
conglomerations on scales beyond our comprehension. The dark matter
pulled together huge amounts of atoms, creating regions dense enough for
nuclear fusion to ignite again and again, giving birth to stars, more
stars, and eventually galaxies. At least, that’s what the leading theory
says. These galaxies then grew and attracted other newly formed
galaxies into clusters and superclusters, where they danced around one
another, sometimes doing dangerously close fly-bys, sometimes colliding,
sometimes merging. Some of them underwent traumatic processes such as
galaxy harassment, stripping, strangulation, and even cannibalism—things
that sound like they apply more to a violent criminal than to a galaxy.
Edwin Hubble, of the eponymous telescope,
was the first to devise a classification scheme to attempt to make
sense of the variety of nearby galaxies. In the 1920s, Hubble spent
night after night taking pictures of the heavens with the 100-inch
telescope at Mount Wilson Observatory
near Pasadena, Calif. In Hubble’s day, the notion that other galaxies
existed was debatable. But some astronomers theorized that the fuzzy
objects they observed were just that. Hubble grouped his observations of
what were later recognized to be galaxies according to their shape:
elliptical or spiral.
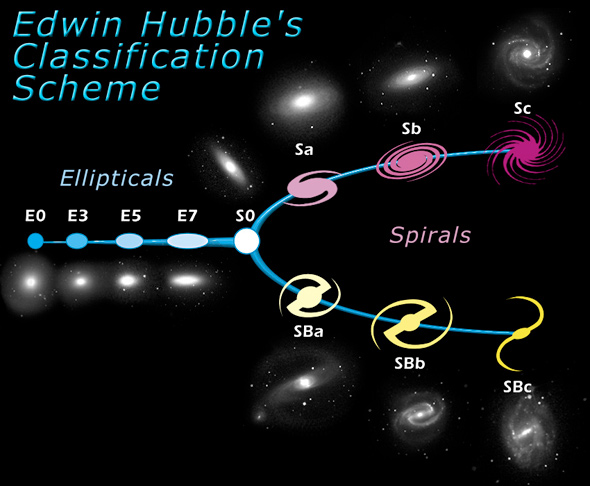
Illustration via HubbleSite News Center
For a long time Hubble’s system seemed to cover all the bases:
Galaxies seemed to slot easily into each category and even into each
subcategory. So easily, in fact, that we thought we had it all figured
out. But with more and more observations, we find there are numerous
galaxies that seem to defy characterization. Hubble somewhat anticipated
this by adding a category for “irregulars.” We now know that the
majority of irregular galaxies are actually interacting galaxies, gravitationally pulling each other apart into all sorts of odd shapes.
We also know there are more to galaxies than just stars. Until the middle of the 20th
century, telescopes that detected visible light were the only game in
town. With data from modern telescopes that collect light across the
electromagnetic spectrum, we now know that in addition to stars,
galaxies contain varying amounts of gas and dust, not to mention plasma,
black holes, superheated jets, shock waves, and much more. The relative
amounts of stars, gas, and dust revealed by infrared, ultraviolet, and X-ray light provide more detailed systems for classifying galaxies.
One such system is based on a galaxy’s color. Galaxies with lots of
gas appear blue because they are dominated by regions of active star
formation and hot, young stars. Galaxies with very little gas and filled
with older, cooler stellar populations appear “red and dead” (yes,
that’s the technical term). Most blue galaxies look like spirals and
most red galaxies are elliptical in shape, therefore spirals are young
galaxies and ellipticals are old: Spirals must evolve into ellipticals,
right?
Maybe. Astronomers are studying how spiral galaxies could run out of
gas, literally and figuratively, and turn into ellipticals. Exactly how
this transformation takes place is still a mystery. Does losing its gas
cause a galaxy to become an elliptical? Or does becoming an elliptical
cause it to lose its gas? Which came (or went) first, the gas or the
galaxy?
A major stumbling block for astronomers is that galaxies evolve on
time scales that dwarf a human lifetime, let alone the career of the
average astronomer. Every stunning picture of a galaxy you’ve seen
represents the tiniest fraction of that galaxy’s existence. We have no
way of physically watching a galaxy grow and change over time. To
compensate, we observe as many galaxies as possible in the hopes of
capturing every stage that can happen in a galaxy’s life. You can think
of it like going to Times Square on New Year’s Eve and photographing
every single person in order to extrapolate the phases of human life,
from infant to senior. But while photos taken in Times Square capture
people as they are over a couple hours, photos of the universe record galaxies as they were over billions of years.
Looking out into space is equivalent to looking back in time. While
light travels tremendously fast, it still has to obey the speed limit:
671 million miles per hour. So if we look at a star 671 million miles
away, we see it not as it is now, but as it was an hour ago. We see
everything in the universe as it was in the past, delayed by however
long the light takes to reach us. And for galaxies, that doesn’t mean
hours, it means millions or even billions of years. Our nearest
neighbor, the Andromeda galaxy, is more than 14 quintillion
miles away from Earth. We see Andromeda as it was 2.5 million years
ago. This means our galactic photo album captures not how galaxies look
today, but how they looked at various points in time.

Courtesy of NASA/ESA/Hubble SM4 ERO Team
Another way astronomers attempt to explain how galaxies transform
draws on a correlation between the shape of a galaxy and the density of
the galaxy’s environment, that is, the number of neighboring galaxies
within a certain distance. It turns out that ellipticals are commonly
located in galaxy clusters while spirals are more likely to be found in
isolation or on the fringes of clusters. This implies that galaxies are
shaped by their surroundings, namely that a high-density environment
could be responsible for quenching star formation and aiding in a
galaxy’s transition from blue to red.
How might star formation be suppressed in practice? This is where
those criminal cosmic acts I mentioned earlier come into play: galaxy
harassment, stripping, strangulation, and cannibalism.
Galaxies in groups, clusters, and superclusters are constantly
interacting via gravity, with the strength of their interactions driven
by their relative sizes, motions, and distances from each other.
Galaxies harass one another gravitationally in high-speed fly-bys and head-on collisions, each distorting the other’s structure into unrecognizable shapes.
Stripping primarily affects spirals “falling” face on
toward the center of a cluster. The motion essentially acts as a wind,
blowing the gas and dust within the galaxy in the opposite direction of
its path, stripping it of fuel for ongoing star formation.
Strangulation occurs when galaxies fall edge first, rather than disk
first. Stars, gas, and dust are pulled out in elongated structures both
toward and away from the cluster. With the galaxy’s gas and dust dispersed, it’s much harder for star formation to continue.
Some galaxies take things to the extreme, ripping smaller galaxies
apart entirely and accreting their stars and gas, effectively
cannibalizing them. The Milky Way is such a cannibal.*
The role of dark matter in shaping the evolution of a galaxy is an
entirely separate can of cosmic worms. Some physicists are probing the
nature of the dark matter itself, but many astronomers believe we can
learn just as much about dark matter by investigating its effects on
galaxies. In fact, it was by studying galaxies that we discovered dark
matter in the 1930s, when astronomers observed that galaxies rotate much
faster than Newton’s Law allowed for, at least based on their visible
matter. Current estimates require roughly six times more dark matter
than visible matter to keep a given galaxy from flying apart.
It’s not the dark matter though, that gives us the most trouble when
trying to model the universe; surprisingly, it’s the visible matter. To
see the effect of star formation on galaxies, we need to model them on
the level of individual stars. However, there are also forces occurring
on scales many times the size of individual galaxies. Powerful black holes
in the centers of galaxies heat up huge amounts of gas and dust in
their vicinity and eject them beyond the farthest boundaries of the
galaxy. And supernovas
blast waves of shock-heated gas well beyond their stellar
neighborhoods. While computers grow more powerful every year,
astronomers still struggle to model this range of scales at a high
enough resolution to tell us anything new. On top of that, doubling the
resolution of a simulation requires roughly 16 times more computing
time. Theorists sit in front of their computers enough as it is. We get
around this by simulating isolated pieces of the universe in detail, but
by definition they only reveal pieces of the picture.
So for now, all we have is this rich vocabulary to describe galaxies for the brief time we have been able to observe them. All we have is pictures—static, narrowly framed snapshots—when what we really need is a movie.
*Correction, Feb. 20, 2014: This article originally misstated that astronomers suspect the Milky Way is a cannibal. They're quite sure that it is.
Summer Ash is director of outreach for Columbia University’s astronomy department and the in-house astrophysicist for The Rachel Maddow Show.
0 Comments:
Post a Comment
<< Home